二氧化碳对脑血流自动调节的调控
Regulation of Cerebral Autoregulation by Carbon Dioxide
Lingzhong Meng, Adrian W. Gelb; Regulation of Cerebral Autoregulation by Carbon Dioxide. Anesthesiology 2015; 122:196–205 doi: https://doi.org/10.1097/ALN.0000000000000506
人工校对+DeepL 翻译 摘要
Cerebral autoregulation describes a mechanism that maintains cerebral blood flow stable despite fluctuating perfusion pressure. Multiple nonperfusion pressure processes also regulate cerebral perfusion. These mechanisms are integrated. The effect of the interplay between carbon dioxide and perfusion pressure on cerebral circulation has not been specifically reviewed. On the basis of the published data and speculation on the aspects that are without supportive data, the authors offer a conceptualization delineating the regulation of cerebral autoregulation by carbon dioxide. The authors conclude that hypercapnia causes the plateau to progressively ascend, a rightward shift of the lower limit, and a leftward shift of the upper limit. Conversely, hypocapnia results in the plateau shifting to lower cerebral blood flows, unremarkable change of the lower limit, and unclear change of the upper limit. It is emphasized that a sound understanding of both the limitations and the dynamic and integrated nature of cerebral autoregulation fosters a safer clinical practice.
脑血流自身调节描述了一种在脑灌注压波动的情况下保持脑血流稳定的机制。多种灌注压以外的过程也调节着脑灌注。是整合机制。二氧化碳和灌注压之间的相互作用对脑循环的影响还没有专门做过综述。根据已发表的数据以及尚无数据支持的某些领域的推测,作者就二氧化碳对脑血流自身调节的调控做出了概念性描述。作者的结论是:高碳酸血症导致脑血流自身调节曲线的平台逐渐抬升,下限右移,上限左移。相反,低碳酸血症导致平台下移至更低的脑血流水平,下限变化不明显,而上限变化尚不清楚。强调了,对脑血流自身调节的局限性、动态性以及整合特性的正确理解,能促进更安全的临床实践。
序言
Cerebral blood flow (CBF) is tightly controlled to meet the disproportionately high metabolic rate of the brain and to wash out the large amount of metabolic wastes thus produced. Multiple physiological processes are engaged in the regulation of CBF. Cerebral autoregulation is a mechanism that maintains a stable CBF for a given magnitude of cerebral metabolic rate in spite of fluctuation of cerebral perfusion pressure (CPP). The original conceptualization was proposed by Lassen who, in 1959, insightfully took the data from 11 groups of subjects reported in 7 different studies and drew the first plot. Lassen’s approach was carefully reviewed and critiqued., Nonetheless, cerebral autoregulation is regularly referenced in clinical practice to guide arterial blood pressure management in both neurological and non-neurological patients, with or without increased intracranial pressure.
脑血流(CBF)受到严格控制,以满足大脑不成比例的高代谢率,并冲走由此产生的大量代谢废物。多个生理过程参与 CBF 的调节。脑血流自身调节是一种在一定程度的脑代谢率下尽管脑灌注压(CPP)有波动仍保持 CBF 稳定的机制。 最初的概念是由 Lassen 提出的,他在 1959 年从 7 项不同研究中报告的 11 组研究对象的数据洞察到,并绘制了第一张图表。拉森的方法被仔细审查和评论。, 尽管如此,脑血流自身调节在临床实践中经常被引用,以指导无论是否有颅内压升高的神经疾病和非神经疾病患者的动脉血压管理。
Cerebral autoregulation is visualized as a correlation plot of CBF (axis of ordinate) against CPP (axis of abscissas) ([fig. 1]). The three key elements of the autoregulation curve are (1) the lower limit, (2) the upper limit, and (3) the plateau. The lower and upper limits are the two sharp inflection points indicating the boundary of pressure-independent flow (the plateau) and the start of pressure-passive flow. The most quoted numbers are the lower limit (CPP) = 60 mmHg, the upper limit (CPP) = 150 mmHg, and the plateau (CBF) = 50 ml/min per 100 g. It needs to be pointed out that these numbers are the means of various groups of subjects in the studies, without any note of the range of distribution or SD., For an individual patient, these means may either underestimate or overestimate the true values. Indeed, it was cautioned by Drummond that there are enormous interindividual and study-to-study variations in the lower limit. The very wide range of the distribution of the lower limit, 40 to 110 mmHg, can be recognized not only in young and healthy volunteers but also in cardiac patients undergoing cardiopulmonary bypass with α-stat acid–base management. The position of the lower limit also depends on the mechanism of hypotension. For example, Fitch et al. showed in baboons that during hemorrhagic hypotension, the lower limit resided at a mean arterial pressure (MAP) that was 65% of the baseline value, whereas during drug-induced hypotension (halothane alone, halothane plus trimetaphan, and halothane plus nitroprusside), the lower limit shifted to a lower MAP that was 35 to 40% of the baseline value.
大脑血流自身调节图像上看是 CBF(纵坐标轴)与 CPP(横坐标轴)的关系图([图 1])。自动调节曲线的三个关键要素是(1)下限,(2)上限,和(3)平台。下限和上限是两个尖锐的拐点,代表着血流与压力无关(平台)到血流开始随压力而变化的交界处。最常引用的数值分别为下限(CPP)=60 mmHg,上限(CPP)=150 mmHg,平台(CBF)=50 ml/(min・100g)。 需要指出的是,这些数字是研究中各组对象的平均值,未见有分布范围或 SD。事实上,Drummond 告诫说,个体间和各研究间的下限存在巨大的差异。下限的分布范围很广,从 40 到 110mmHg,不仅在年轻健康的志愿者中可以确认,而且在接受心肺分流和 α 稳态酸碱管理的心脏病患者中也可以确认。下限的位置也取决于低血压的机制。例如,Fitch 等人在狒狒身上显示,在出血性低血压期间,下限停留在平均动脉压(MAP)为基线值的 65%,而在药物诱导的低血压期间(单用氟烷、氟烷加曲美坦、氟烷加硝普钠),下限转移到平均动脉压为基线值的 35-40%。
图 1
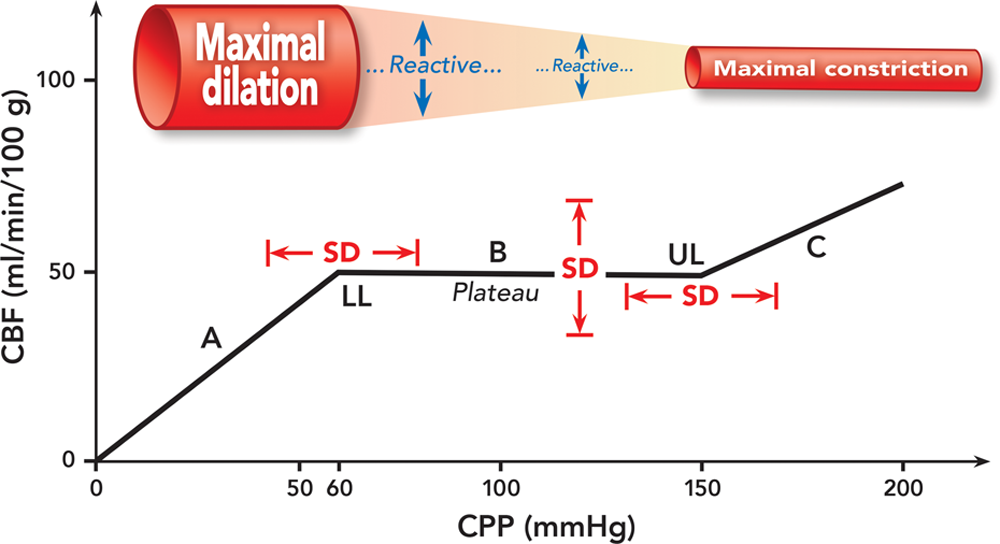
Fig. 1. Cerebral autoregulation is visualized as a correlation plot between cerebral blood flow (CBF) and cerebral perfusion pressure (CPP). CBF remains stable between the lower limit (LL) and the upper limit (UL) (portion B, plateau). CBF is pressure passive at the CPP range below the lower limit (portion A) and above the upper limit (portion C). This illustration uses a CPP of 60 mmHg as the lower limit, a CPP of 150 mmHg as the upper limit, and a CBF of 50 ml/min per 100 g as the plateau. However, these regularly quoted numbers are not fixed; rather, they vary interindividually and intraindividually depending on a variety of factors. Therefore, we take a note of SD to emphasize that these parameters have a wide range of distribution. The cerebrovascular reactivity is also illustrated.
图 1. 脑血流(CBF)和脑灌注压(CPP)的相关图展示脑血流自身调节。CBF 在下限(LL)和上限(UL)之间保持恒定(B 部分,平台)。CBF 在低于下限(A 部分)和高于上限(C 部分) 的 CPP 范围内是随压力变化的。本图以 60mmHg 的 CPP 为下限,150mmHg 的 CPP 为上限,50ml/min/100g 的 CBF 为平台。然而,这些经常引用的数值并非固定不变;相反,各种因素可使这些数值因人而异且同一个体也不总一样。因此,我们标注上 SD,强调这些参数的值分布范围较大。脑血管的反应性也一并图示。
The execution of cerebral autoregulation relies on the robust cerebrovascular reactivity that engenders dilation to a decrease in CPP and constriction to an increase in CPP (fig. 1). However, cerebrovascular reactivity is not exclusively linked to CPP. Changes in other physiological processes, notably, carbon dioxide, can also alter cerebral vasomotor tone and thus regulate CBF. Intuitively, perfusion pressure and nonperfusion pressure CBF-regulating processes interact and integrate at the point of cerebrovascular resistance regulation; thus, the effect of the interplay of distinct processes on CBF may differ to a stand-alone process. Therefore, cerebral autoregulation should be regarded as a mobile instead of a fixed plot when nonperfusion pressure processes are also involved. Ignorance of this dynamic and integrative nature of CBF regulation can be risky in clinical practice because the position and shape of the autoregulation curve may have shifted away from what we normally assume. Although the above cited numbers may apply in some young, healthy, normotensive, nonanesthetized, and resting subjects and when perfusion pressure is the only process engaged in CBF regulation, they may not be suitable in other circumstances. We emphasize that the autoregulation curve conceived by Lassen is not a one-size-fits-all phenomenon; rather, its position and shape may change following changes in pertinent physical, medical, neurological, or physiological conditions. Therefore, proper arterial blood pressure management in the effort of CBF optimization can only be fulfilled when the influences of nonperfusion pressure processes on cerebral autoregulation are appreciated.
大脑血流自身调节发挥作用有赖于强大的脑血管反应性,在 CPP 下降时扩张,在 CPP 增加时收缩(图 1)。然而,脑血管反应性并不完全与 CPP 有关。其他生理过程的变化,特别是二氧化碳的变化,也可以改变脑血管舒缩的张力,从而调节 CBF。直观地说,灌注压和灌注压之外的 CBF 调节过程在脑血管阻力调节上相互作用和整合;因此,不同过程的相互作用对 CBF 的影响可能与单独过程的不同。因此,当灌注压之外的过程也参与其中时,大脑血流自身调节应被视为一个变动的而不是固定的情节。忽视 CBF 调节的这种动态和整合特性在临床实践中可能是有风险的,因为自动调节曲线的位置和形状可能已经偏离了我们通常的假设。尽管上述数字可能适用于一些年轻、健康、血压正常、非麻醉和静息状态的受试者,并且当灌注压是参与 CBF 调节的唯一过程时,这些数值不适用于其他情况。我们强调,Lassen 设想的自动调节曲线不是一个万能的现象;相反,它的位置和形状可能会随着身体、医疗、神经或生理条件的相关变化而改变。因此,只有认识到非灌注压过程对大脑血流自身调节的影响,才能在优化 CBF 中达到最佳动脉血压管理。
Carbon dioxide is a known powerful modulator of cerebral vasomotor tone, and change in arterial blood carbon dioxide partial pressure (PaCO2) is frequently encountered in clinical care., The influence of carbon dioxide on cerebral autoregulation has not been specifically reviewed. A sound understanding of this topic facilitates clinical decision making and promotes a culture of “safe” practice. On the basis of the published large animal and human data and some speculation on the components that are without direct data support, we present a detailed discussion of the integrated effect of carbon dioxide and perfusion pressure on the cerebral circulation.
二氧化碳是已知的脑血管舒缩张力的强大调节器,而动脉血二氧化碳分压(PaCO2)的变化在临床救治中经常遇到。, 二氧化碳对脑血流自身调节的影响还没有专门的综述。对这一主题的正确理解有助于临床决策和促进医疗 "安全" 文化。在已发表的大型动物和人类数据以及一些没有直接数据支持的内容的推测的基础上,我们对二氧化碳和灌注压对脑循环的综合影响进行了详细讨论。
高碳酸血症对脑部自动调节的影响
Effect of Hypercapnia on Cerebral Autoregulation
Hypercapnia increases CBF by cerebral vasodilation. Thus, there are two pertinent queries when considering its effect on cerebral autoregulation. The first is how it affects the lower limit. The combined vasodilatory effects imposed by hypotension and hypercapnia could shift the lower limit rightward. The other query is how it affects the upper limit. The dilation induced by hypercapnia could adversely affect the hypertension-induced constriction, rendering a leftward shift of the upper limit.
高碳酸血症通过脑血管扩张增加 CBF。因此,在考虑其对脑血流自身调节的影响时,有两个相关的问题。首先是它是如何影响下限的。低血压和高碳酸血症造成的血管扩张效应可能使下限向右移动。另一个问题是它是如何影响上限的。高碳酸血症引起的扩张可能对高血压引起的收缩产生不利影响,使上限向左移动。
Harper in 1966 was among the earliest to show in dogs that the lower limit was lost and the pressure–flow relationship became linear during severe hypercapnia (PaCO2 = 70 to 90 mmHg) compared with normocapnia (PaCO2 = 30 to 40 mmHg). Also in dogs, Häggendal and Johansson showed that the lower limit occurred at an MAP of 50 to 60 mmHg under normocapnia (PaCO2 = 30 to 50 mmHg); however, during hypercapnia (PaCO2 = 70 to 95 mmHg), the lower limit shifted to a much higher MAP of 80 to 100 mmHg. Later, Raichle and Stone demonstrated in monkeys that the lower limit was shifted upward and rightward and ultimately abolished by acutely increasing PaCO2 in a stepwise manner (PaCO2 = 33, 48, 57, and 70 mmHg, respectively).
1966 年,Harper 最早在狗身上发现,与正常碳酸血症(PaCO2=30 至 40 mmHg)相比,在严重高碳酸血症(PaCO2=70 - 90 mmHg)时,下限消失,压力 - 流量关系变为线性。同样在狗身上,Häggendal 和 Johansson 表明,二氧化碳正常(PaCO2=30 - 50mmHg)时,低限发生在 MAP 为 50 - 60mmHg 时;然而,在二氧化碳分压较高(PaCO2=70 至 95mmHg)时,低限转移到 80 -100mmHg 的更高 MAP。后来,Raichle 和 Stone 在猴子身上证明,下限向上和向右移动,通过急性逐步快速增加 PaCO2(分别为,PaCO2=33、48、57 和 70 mmHg)而最终上下限消失。
Regarding the upper limit, Ekström-Jodal et al. showed in dogs that autoregulation was conserved until 225 mmHg (MAP) during normocapnia; however, during moderate hypercapnia (PaCO2 = 40 to 60 mmHg), the autoregulatory pressure range was shorter, with an upper limit of 150 mmHg, and during more marked hypercapnia (PaCO2 >60 mmHg), the upper limit was as low as 125 mmHg.
关于上限,Ekström-Jodal et al. 在狗身上显示,在正常碳酸血症期间,自动调节功能一直保持到 225 mmHg(MAP);然而,在中度高碳酸血症期间(PaCO2 = 40 至 60 mmHg),自动调节压力范围更短,上限为 150 mmHg,在更明显的高碳酸血症期间(PaCO2 > 60 mmHg),上限低至 125 mmHg。
In human subjects, McCulloch et al. found that the threshold at which hypercapnia significantly impaired autoregulation averaged 56 mmHg (PaCO2) during sevoflurane anesthesia and 61 mmHg during propofol anesthesia. In patients undergoing cardiopulmonary bypass, Murkin et al. showed that α-stat acid–base management preserved, whereas pH-stat (carbon dioxide supplementation) impaired autoregulation. In ventilated very low-birth-weight infants, Kaiser et al. found that autoregulation was progressively impaired and cerebral perfusion became progressively pressure passive with escalating hypercapnia. However, none of these human studies examined in detail how the plateau, the lower limit, and the upper limit are affected by hypercapnia.[^18–20]
在人类受试者中,McCulloch et al. 发现,在七氟醚麻醉期间,高碳酸血症明显损害自动调节的阈值平均为 56 mmHg(PaCO2),在丙泊酚麻醉期间为 61 mmHg。在接受心肺分流的病人中,Murkin et al. 显示,α-stat 酸碱管理保留了自动调节,而 pH-stat(二氧化碳补充)会损害自动调节。Kaiser et al. 在机械通气的极低出生体重的婴儿中发现,随着高碳酸血症的增加,自动调节功能逐渐受损,脑灌注逐渐变为随压力而变动。然而,这些人类研究都没有详细研究平台、下限和上限如何受到高碳酸血症的影响。
In light of these studies, we propose the following construct as illustrated in figure 2 to explain the effect of hypercapnia on cerebral autoregulation. The ensuing discussion in this section is based on this figure unless specified otherwise. The plateau shifts upward during hypercapnia due to the cerebral vasodilation being induced.
根据这些研究,我们提出了以下概念,如 图 2 所示,以解释高碳酸血症对大脑血流自身调节的影响。除非另有说明,本节接下来的讨论以该图为基础。在高碳酸血症期间,由于诱发了脑血管扩张,平台会向上移动。
图 2
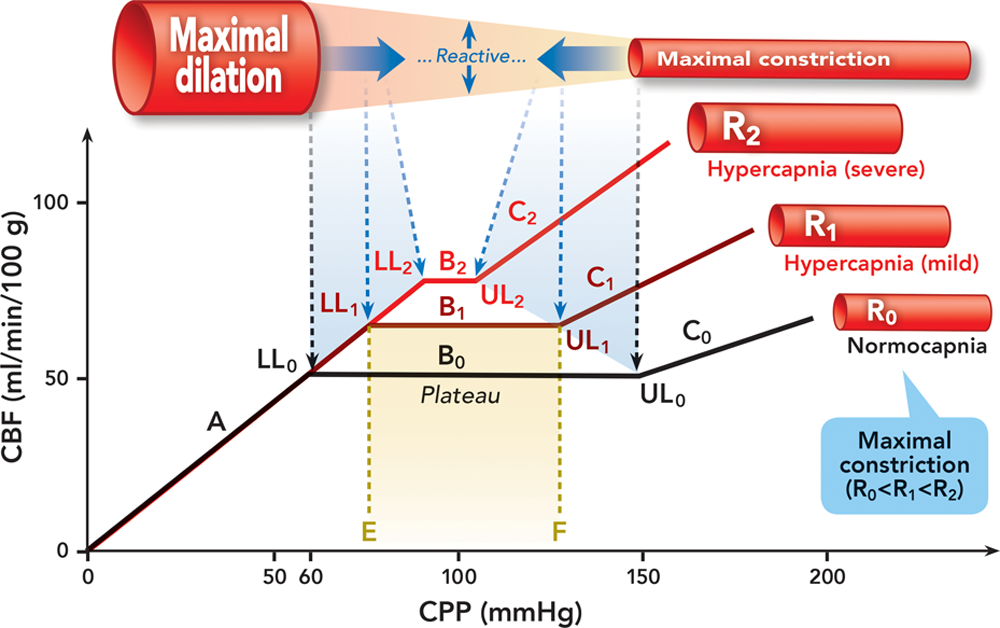
Fig. 2. Effect of hypercapnia on cerebral autoregulation. Autoregulation curves are in black at normocapnia and red at hypercapnia. Cerebral resistance vessels are illustrated in red/pink. The bold solid blue arrows indicate the dynamic shift of the maximally dilated and constricted cerebral resistance vessels at hypercapnia. The dashed black and blue lines/arrows indicate the lower and upper limits at normocapnia and hypercapnia, respectively. A = the curve below the lower limit; B = the plateau at normocapnia (B0), mild hypercapnia (B1), and severe hypercapnia (B2); C = the curve above the upper limit at normocapnia (C0), mild hypercapnia (C1), and severe hypercapnia (C2); CBF = cerebral blood flow; CPP = cerebral perfusion pressure; LL = the lower limit at normocapnia (LL0), mild hypercapnia (LL1), and severe hypercapnia (LL2); R = calibers of cerebral resistance vessels at normocapnia (R0), mild hypercapnia (R1), and severe hypercapnia (R2); UL = the upper limit at normocapnia (UL0), mild hypercapnia (UL1), and severe hypercapnia (UL2).
图 2. 高碳酸血症对大脑血流自身调节的影响。自身调节曲线在正常碳酸血症为黑色,在高碳酸血症为红色。大脑阻力血管以红色 / 粉红色表示。蓝色实心粗大箭头表示高碳酸血症时脑阻力血管最大程度扩张和收缩的的动态变化。黑色和蓝色的虚线 / 箭头分别表示正常和高碳酸血症时的下限和上限。A = 低于下限的曲线部分;B = 正常碳酸血症(B0)、轻度高碳酸血症(B1)和严重高碳酸血症(B2)时的平台;C = 正常碳酸血症(C0)、轻度高碳酸血症(C1)和严重高碳酸血症(C2)时高于上限的曲线部分;CBF = 脑血流量;CPP = 大脑灌注压。LL = 正常碳酸血症(LL0),轻度高碳酸血症(LL1),和严重高碳酸血症(LL2)时的下限;R = 正常碳酸血症(R0),轻度高碳酸血症(R1),和严重高碳酸血症(R2)时的脑阻力血管的口径;UL = 正常时的脑阻力血管的口径(UL0),轻度高碳酸血症(UL1),和严重高碳酸血症(UL2)时的上限。
When CPP is decreasing, cerebral resistance vessels dilate until the lower limit is reached. However, with hypercapnia-induced dilation, maximal dilation and therefore the lower limit is reached at a higher CPP during hypercapnia than normocapnia, that is, the lower limit is shifted rightward. For example, at point E on the CPP axis at normocapnia, cerebral resistance vessels are not maximally dilated yet because the CPP is higher than the lower limit; however, for the same CPP at hypercapnia, cerebral resistance vessels are already maximally dilated due to the additional dilation imposed by hypercapnia. We overlap the portions of the autoregulation curve below the lower limit based on the premise that the calibers of the maximally dilated cerebral resistance vessels at hypercapnia and normocapnia are the same. This premise is plausible if there is truly a fixed size limit to which cerebral resistance vessels can dilate. Otherwise, a different construct would ensue.
当 CPP 下降时,大脑阻力血管会扩张,直到达到下限。然而,对于高碳酸血症引起的扩张,在高碳酸血症期间,比在正常碳酸血症时更高的 CPP 就会出现最大程度的血管扩张并因此触及下限,也就是说,下限是向右移的。例如,在常态下 CPP 轴上的 E 点,由于 CPP 高于下限,大脑阻力血管还没有达到最大扩张;然而,在高碳酸血症时,由于高碳酸血症引起额外扩张,同样的 CPP,大脑阻力血管已经达到最大程度扩张了。我们将自动调节曲线低于下限的部分重叠,前提是高碳酸血症和正常碳酸血症时最大程度扩张的脑阻力血管的口径相同。如果真的存在一个固定尺寸限制着大脑阻力血管可以扩张的程度,作为假设是合理的。否则,就会产生不同的概念。
When CPP is increasing, cerebral resistance vessels constrict until the upper limit is reached. However, at hypercapnia, the upper limit is reached at a lower CPP than normocapnia, that is, the upper limit is shifted leftward due to the antagonism of the hypertension-induced vasoconstriction by the hypercapnia-induced vasodilation. For example, at point F on the CPP axis at normocapnia, cerebral resistance vessels are not maximally constricted yet because the CPP is lower than the upper limit; however, for the same CPP at hypercapnia, they are already maximally constricted due to the case that further constriction is negated by hypercapnia. Because different degrees of hypercapnia exert differing strengths of dilation, the calibers of the maximally constricted cerebral resistance vessels are different at differing severity of hypercapnia. As a consequence, the portion of the autoregulation curve above the upper limit at hypercapnia does not overlap with normocapnia and has a steeper slope.
当 CPP 增加时,大脑阻力血管会收缩,直到达到上限。然而,在高碳酸血症时,达到上限的 CPP 要比正常低,也就是说,由于高碳酸血症引起的血管扩张对高血压引起的血管收缩的拮抗作用,上限向左移动了。例如,在常态下 CPP 轴上的 F 点,由于 CPP 低于上限,大脑阻力血管还没有最大限度地收缩;然而,在高碳酸血症时,由于高碳酸血症阻止了血管进一步收缩,相同的 CPP 下,血管已经最大限度收缩。由于不同程度的高碳酸血症会产生不同的扩张强度,在不同严重程度的高碳酸血症下,最大收缩的脑阻力血管的口径也不同。因此,高碳酸血症时自动调节曲线上限以上的部分与正常碳酸血症不重叠,而且斜率较陡。
In summary, during hypercapnia, the plateau of the autoregulation curve is shifted upward and shortened, the lower limit is shifted rightward, and the upper limit is shifted leftward. The extent of these changes depends on the severity of hypercapnia. At severe hypercapnia when cerebral resistance vessels are maximally dilated, the plateau is lost and the pressure–flow relationship is linear.
总之,在高碳酸血症期间,自动调节曲线的平台向上移动并缩短,下限向右移动,而上限向左移动。这些变化的程度取决于高碳酸血症的严重程度。在严重的高碳酸血症时,当大脑阻力血管最大限度地扩张时,平台反应消失,压力 - 流量关系呈线性。
低碳酸血症对大脑血流自身调节的影响
Effect of Hypocapnia on Cerebral Autoregulation
Hypocapnia decreases CBF by cerebral vasoconstriction. As such, there are two pertinent queries relating to its effect on cerebral autoregulation. The first is how the lower limit moves because the effects of hypotension and hypocapnia on cerebral resistance vessels are opposite; one dilates and the other constricts. The other query is how the upper limit shifts because both hypertension and hypocapnia induce cerebral vasoconstriction.
低碳酸血症通过脑血管收缩使 CBF 减少。因此,有两个与它对大脑血流自身调节的影响有关的问题。首先是下限是如何移动的,因为低血压和低碳酸血症对脑阻力血管的影响是相反的;一个扩张,另一个收缩。另一个疑问是上限如何移动,因为高血压和低碳酸血症都会诱发脑血管收缩。
In 1965, Harper and Glass showed by bleeding dogs that when MAP was reduced to 100 mmHg from a baseline of 150 mmHg, the CBF response to hypocapnia persisted but was much reduced, and when MAP was further reduced to 50 mmHg, the CBF response to hypocapnia was lost. They proposed an “over-ride” mechanism to explain their finding by theorizing that responding to tissue ischemia and hypoxia takes precedence over the maintenance of tissue carbon dioxide homeostasis. Work conducted by Whitelaw et al. in newborn piglets subjected to bleeding showed that hypocapnia failed to further decrease CBF when MAP was reduced to 38 mmHg or less even though it could produce a substantial decrease in CBF at an MAP of 45 mmHg or above.
1965 年,Harper 和 Glass 通过给狗放血表明,当 MAP 从 150mmHg 的基线降低到 100mmHg 时,CBF 对低碳酸血症的反应持续存在,但大大降低;当 MAP 进一步降低到 50mmHg 时,CBF 对低碳酸血症的反应消失。他们提出了一种 "覆盖" 机制来解释他们的发现,认为对组织缺血和缺氧的反应优先于维持组织的二氧化碳平衡。惠特洛等人给试验小猪仔放血显示,当 MAP 降低到 38 mmHg 或 更低时,低碳酸血症未能进一步降低 CBF,尽管它在 MAP 为 45 mmHg 或更高时能产生 CBF 的大幅下降。
In the context of drug-induced hypotension, serial works by Artru et al. showed that the cerebrovascular response to hypocapnia was abolished in dogs during hypotension to an MAP of 50 mmHg induced with sodium nitroprusside,, trimethaphan, and nitroglycerin. In contrast, Matta et al. showed that the cerebrovascular response to hypocapnia was attenuated, but not abolished, during nitroprusside-induced hypotension (MAP = 60 mmHg) in patients anesthetized with isoflurane. Similarly, Endoh et al. showed that nicardipine, nitroglycerin, and prostaglandin E1-induced hypotension (MAP = 55 to 60 mmHg) attenuated, but did not abolish, the cerebrovascular hypocapnia response in patients anesthetized with propofol and fentanyl.
在药物诱导的低血压方面,Artru et al. 的系列工作表明,在用硝普钠、、三甲胺、和硝酸甘油诱导的低血压达到 50 mmHg 时,狗的脑血管对低碳酸血症的反应消失了。 相反,Matta et al. 显示,在用异氟醚麻醉的病人中,硝普钠诱导的低血压(MAP = 60 mmHg)期间,脑血管对低碳酸的反应减弱,但没有消失。同样,Endoh et al. 显示,尼卡地平、硝酸甘油和前列腺素 E1 诱导的低血压(MAP = 55 至 60 mmHg)减弱了丙泊酚和芬太尼麻醉患者的脑血管低碳酸血症反应,但没有废除。
With regard to the hypotension induced by anesthetic agents, Okuda et al. showed that the cerebrovascular hypocapnia response was abolished at an MAP of 45 mmHg induced by halothane in baboons. Artru et al. showed in dogs that the cerebrovascular response to hypocapnia was partially preserved during isoflurane-induced hypotension to an MAP of 50 mmHg. In patients, Matta et al. found that the cerebrovascular carbon dioxide reactivity was attenuated during isoflurane-induced hypotension as low as 60 mmHg (MAP).
关于麻醉剂引起的低血压,Okuda et al. 表明,在氟烷引起的 MAP 为 45mmHg 时,狒狒的脑血管低碳酸血症反应消失。Artru et al. 在狗身上显示,在异氟烷诱导的低血压达到 50 mmHg 时,脑血管对低碳酸血症的反应部分得以保留。在病人身上,Matta et al. 发现,在异氟烷诱导的低血压低至 60 mmHg(MAP)时,脑血管的二氧化碳反应性减弱。
Collectively, there is ample evidence demonstrating that the cerebrovascular reactivity to hypocapnia is significantly attenuated or abolished during hemorrhage-, drug-, or anesthesia-induced hypotension. However, these studies do not directly tackle the question of how hypocapnia affects cerebral autoregulation. The effect of hypotension on cerebrovascular hypocapnia reactivity and effect of hypocapnia on cerebrovascular pressure reactivity, that is, autoregulation, are related but distinctive issues. The former is typically studied via investigating the change in CBF following a change in PaCO2 under hypotensive condition, whereas the latter via investigating the change in CBF following a change in CPP at hypocapnia. Surprisingly, there is scant evidence directly addressing the latter issue. A study conducted by Artru et al. examined the effect of hypocapnia on the lower limit while the CPP was gradually decreased via hemorrhage in dogs. They found that hypocapnia did not cause a substantial shift of the lower limit which was at 61% of baseline CPP at hypocapnia and 59% of baseline CPP at normocapnia, and that the slopes of the autoregulation curve below the lower limit did not significantly differ between hypocapnia and normocapnia.
总之,有大量证据表明,在出血、药物或麻醉引起的低血压期间,脑血管对低碳酸的反应性明显减弱或消失。 然而,这些研究没有直接解决低碳酸血症如何影响大脑血流自身调节的问题。低血压对脑血管低碳酸血症反应性的影响和低碳酸血症对脑血管压力反应性的影响,即自动调节,是相关但不同的问题。前者通常是通过调查低血压条件下 PaCO2 变化后 CBF 的变化来研究的,而后者则是通过调查低碳酸血症时 CPP 变化后 CBF 的变化。令人惊讶的是,很少有证据直接解决后一个问题。Artru et al. 进行的一项研究通过给狗放血令 CPP 逐渐降低,来考察低碳酸血症对下限的影响。他们发现,低碳酸血症并没有导致下限的实质性移动,低碳酸血症时下限为基线 CPP 的 61%,正常碳酸血症时下限为基线 CPP 的 59%,而且下限以下的自动调节曲线的斜率在低碳酸血症和正常碳酸血症之间没有明显区别。
In light of these considerations, we propose the constructs illustrated in figures 3 and 4 to describe the effect of hypocapnia on cerebral autoregulation. The constructs in figures 3 and 4 are based on the distinct speculation on the effect of hypocapnia on the upper limit. The ensuing discussion in this section is based on these two figures unless specified otherwise. The plateau descends to a lower CBF with hypocapnia due to cerebral vasoconstriction.
鉴于这些考虑,我们提出了图 3 和 4 中的概念示例,来描述低碳酸血症对大脑血流自身调节的影响。图 3 和图 4 中的概念是基于对低碳酸血症对上限影响的明显推测。除非另有说明,本节接下来的讨论以这两个图为基础。由于脑血管收缩,平台在低碳酸血症时下降到较低的 CBF。
图 3
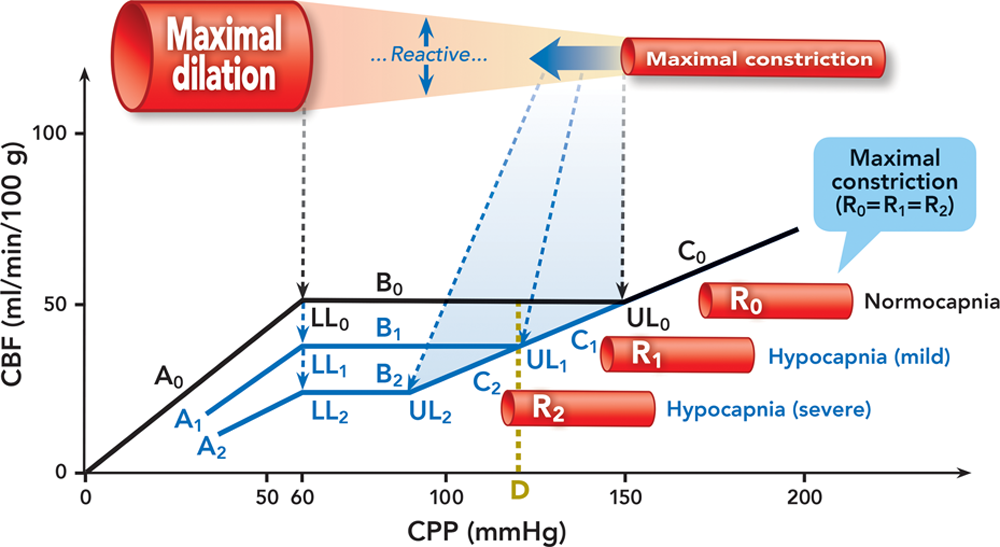
Fig. 3. Effect of hypocapnia on cerebral autoregulation. We speculate that the calibers of the maximally constricted cerebral resistance vessels at normocapnia and hypocapnia are the same. Autoregulation curves are in black at normocapnia and blue at hypocapnia. Cerebral resistance vessels are illustrated in red/pink. The bold solid blue arrow indicates the dynamic shift of the maximally constricted cerebral resistance vessels at hypocapnia. The dashed black and blue lines/arrows indicate the lower and upper limits at normocapnia and hypocapnia, respectively. A = the curve below the lower limit at normocapnia (A0), mild hypocapnia (A1), and severe hypocapnia (A2); B = the plateau at normocapnia (B0), mild hypocapnia (B1), and severe hypocapnia (B2); C = the curve above the upper limit at normocapnia (C0), mild hypocapnia (C1), and severe hypocapnia (C2); CBF = cerebral blood flow; CPP = cerebral perfusion pressure; LL = the lower limit at normocapnia (LL0), mild hypocapnia (LL1), and severe hypocapnia (LL2); R = calibers of cerebral resistance vessels at normocapnia (R0), mild hypocapnia (R1), and severe hypocapnia (R2); UL = the upper limit at normocapnia (UL0), mild hypocapnia (UL1), and severe hypocapnia (UL2).
图 3. 低碳酸血症对大脑血流自身调节的影响。我们推测,正常血压和低压时,最大收缩的大脑阻力血管的口径是一样的。自身调节曲线在正常碳酸血症时为黑色,在低碳酸血症时为蓝色。大脑阻力血管以红色 / 粉红色表示。蓝色实心粗大箭头表示低碳酸血症时最大收缩的脑阻力血管的动态变化。黑色和蓝色的虚线 / 箭头分别表示正常和度和低碳酸血症时的下限和上限。A = 正常碳酸血症(A0)、轻度低碳酸血症(A1)和重度低碳酸血症(A2)时低于下限的曲线;B = 正常碳酸血症(B0)、轻度低碳酸血症(B1)和重度低碳酸血症(B2)时的平台;C = 正常碳酸血症(C0)、轻度低碳酸血症(C1)和重度低碳酸血症(C2)时高于上限的曲线;CBF = 大脑血流量。CPP = 脑灌注压;LL = 正常碳酸血症时的下限(LL0),轻度碳酸血症(LL1)和重度碳酸血症(LL2);R = 正常碳酸血症时的脑阻力血管口径(R0),轻度碳酸血症(R1)和重度碳酸血症(R2);UL = 正常碳酸血症时的上限(UL0),轻度碳酸血症(UL1)和重度碳酸血症(UL2)。
图 4
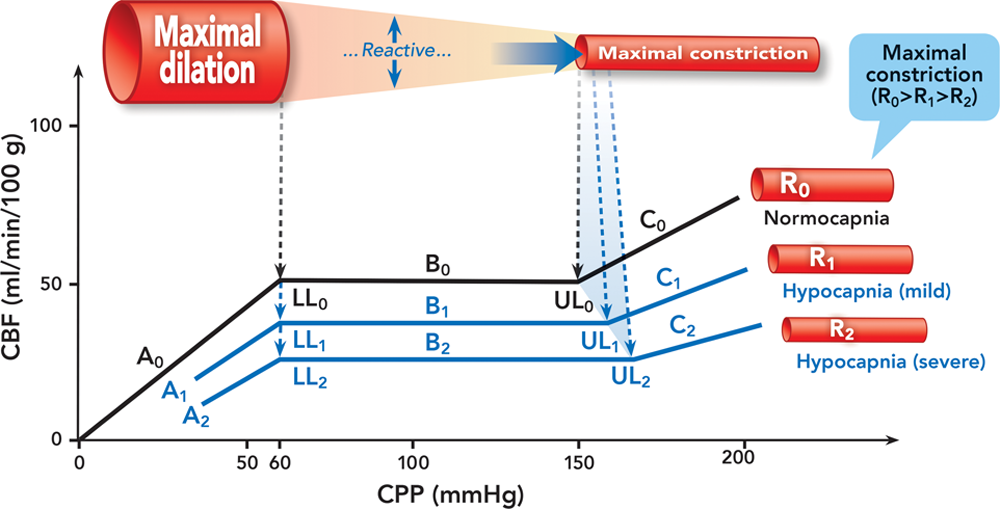
Fig. 4. Effect of hypocapnia on cerebral autoregulation. We speculate that the caliber of the maximally constricted cerebral resistance vessels at hypocapnia is smaller than normocapnia and assume that hypocapnia causes a rightward shift of the upper limit. Autoregulation curves are in black at normocapnia and blue at hypocapnia. Cerebral resistance vessels are illustrated in red/pink. The bold solid blue arrow indicates the dynamic shift of the maximally constricted cerebral resistance vessels at hypocapnia. The dashed black and blue lines/arrows indicate the lower and upper limits at normocapnia and hypocapnia, respectively. A = the curve below the lower limit at normocapnia (A0), mild hypocapnia (A1), and severe hypocapnia (A2); B = the plateau at normocapnia (B0), mild hypocapnia (B1), and severe hypocapnia (B2); C = the curve above the upper limit at normocapnia (C0), mild hypocapnia (C1), and severe hypocapnia (C2); CBF = cerebral blood flow; CPP = cerebral perfusion pressure; LL = the lower limit at normocapnia (LL0), mild hypocapnia (LL1), and severe hypocapnia (LL2); R = calibers of cerebral resistance vessels at normocapnia (R0), mild hypocapnia (R1), and severe hypocapnia (R2); UL = the upper limit at normocapnia (UL0), mild hypocapnia (UL1), and severe hypocapnia (UL2).
图 4. 低碳酸血症对大脑血流自身调节的影响。我们推测,在低碳酸血症时,最大收缩的脑阻力血管的口径比正常血症时小,并假设低碳酸血症会导致上限的右移。常态下自动调节曲线为黑色,低碳酸血症时为蓝色。大脑阻力血管以红色 / 粉红色表示。蓝色实心粗大箭头表示低碳酸血症时最大收缩的脑阻力血管的动态变化。黑色和蓝色的虚线 / 箭头分别表示正常和低碳酸血症时的下限和上限。A = 正常碳酸血症(A0)、轻度低碳酸血症(A1)和重度低碳酸血症(A2)时低于下限的曲线;B = 正常碳酸血症(B0)、轻度低碳酸血症(B1)和重度低碳酸血症(B2)时的平台;C = 正常碳酸血症(C0)、轻度低碳酸血症(C1)和重度低碳酸血症(C2)时高于上限的曲线;CBF = 大脑血流量。CPP = 脑灌注压;LL = 正常碳酸血症时的下限(LL0)、轻度碳酸血症(LL1)和重度碳酸血症(LL2);R = 正常碳酸血症(R0)、轻度碳酸血症(R1)和重度碳酸血症(R2)时大脑阻力血管的口径;UL = 正常碳酸血症(UL0)、轻度碳酸血症(UL1)和重度碳酸血症(UL2)时的上限。
To the best of our knowledge, the study by Artru et al. is the only one so far that directly examined the effect of hypocapnia on the lower limit. In accordance with the results in the study by Artru et al., we have kept the position of the lower limit at hypocapnia the same as normocapnia and the slope of the autoregulation curve below the lower limit at hypocapnia not significantly different from normocapnia. Nonetheless, the abundant evidence that the cerebrovascular reactivity to hypocapnia is significantly weakened or lost during hypotension makes the drawing of the autoregulation curve in the proximity of the lower limit during hypocapnia complex. One could argue that the plateau should swing upward when the CPP is critically decreased due to the attenuation of the cerebrovascular hypocapnia response by hypotension. This seems rational when considering that the “over-ride” of the hypocapnia vasoconstrictive effect could have restored the decreased CBF. Indeed, this consideration seems supported by the rabbit data presented by Czosnyka et al. where a smooth upswing of the plateau in the proximity of the lower limit was demonstrated even though it was not clear whether the curve was generated during hypocapnia. However, such speculations suggest that during hypocapnia the CBF at hypotension could be higher than normotension. To date, the available data show that the CBF during combined hypocapnia and hypotension is not higher than that when hypocapnia and normotension are combined., In addition, “over-ride” is a mechanism dealing specifically with the effect of hypotension on cerebrovascular carbon dioxide reactivity that is different to the effect of hypocapnia on cerebrovascular pressure reactivity or autoregulation.
据我们所知,Artru et al. 的研究是迄今为止唯一直接研究低碳酸血症对下限影响的研究。根据 Artru *et al.* 的研究结果,我们将低碳酸血症时的下限位置与正常血症保持一致,低碳酸血症时下限以下的自动调节曲线的斜率与正常血症无明显差异。然而,大量的证据表明,在低血压期间,脑血管对低碳酸血症的反应性明显减弱或消失,这使得在低碳酸血症时在下限附近绘制自动调节曲线变得复杂。由于低血压减弱了脑血管对低碳酸血症的反应,CPP 严重下降时平台应该向上摆动。当考虑到低碳酸血症的血管收缩作用被 "超驰",CBF 的下降有可能恢复,这似乎是合理的。事实上,这种考虑似乎得到了 Czosnyka et al. 提供的兔子数据的支持,其中,尽管不清楚该曲线是否是在低碳酸血症期间绘制的,但在接近下限的地方,平台的平稳上扬得到了证明。然而,这种推测认为,在低碳酸血症期间,低血压时的 CBF 可能比正常血压高。迄今为止,现有的数据显示,低碳酸血症合并低血压时的 CBF 并不比低碳酸血症合并正常血压时的 CBF 高。, 此外,"超驰" 是一种专门处理低血压对脑血管二氧化碳反应性影响的机制,与低碳酸血症对脑血管压力反应性或自动调节作用不同。
How the upper limit is affected by hypocapnia is not clear due to the lack of data. There are two lines of speculation. If we hypothesize that the calibers of the maximally constricted cerebral resistance vessels are the same between hypocapnia and normocapnia, the construct in figure 3 applies. In this case, the upper limit shifts leftward due to the “background” constriction induced by hypocapnia. For example, at point D on the CPP axis at normocapnia, cerebral resistance vessels are not maximally constricted yet. However, for the same CPP at hypocapnia, they are maximally constricted as a result of the additional constriction imposed by hypocapnia. We overlap the portions of the autoregulation curve above the upper limit at hypocapnia and normocapnia. The overlap is plausible in considering that, for a given CPP higher than the upper limit, the CBF at hypocapnia should be the same as normocapnia because the flow resistances determined by the calibers of cerebral resistance vessels are the same.
由于缺乏数据,上限如何受到低碳酸血症的影响尚不清楚。有两种猜测。如果我们假设在低碳酸血症和正常碳酸血症之间,最大收缩的脑阻力血管的口径是相同的,那么图 3 的结构就适用。在这种情况下,由于低碳酸血症引起的 "背景" 收缩,其上限向左移动。例如,在常态下 CPP 轴上的 D 点,大脑阻力血管还没有达到最大收缩。然而,在低碳酸血症时,对于相同的 CPP,由于低碳酸血症引起的额外收缩,它们被最大限度地收缩了。我们将低碳酸血症和正常碳酸血症时的上限以上的自动调节曲线部分重叠起来。考虑到对于高于上限的给定 CPP,低碳酸血症时的 CBF 应与正常碳酸血症相同,因为由脑阻力血管口径决定的血流阻力是相同的,这种重叠是合理的。
The other line of speculation is to hypothesize that the caliber of the maximally constricted cerebral resistance vessels at hypocapnia is smaller than normocapnia due to the extra constriction imposed by hypocapnia. In this case, the construct in figure 4 may apply. The upper limit may or may not shift rightward even though we opt for a rightward shift in this discussion. This line of speculation is shared by Paulson et al. who believed that, at hypocapnia, the upper limit shifted rightward and as a consequence, the plateau was lengthened. However, neither Paulson et al. nor the two studies cited by Paulson et al., specifically studied the effect of hypocapnia on the upper limit. Future research is warranted to address this unknown aspect.
另一种推测是假设在低碳酸血症时,由于低碳酸血症造成的额外收缩,最大收缩的脑阻力血管的口径比正常碳酸血症小。在这种情况下,图 4 的结构可能适用。尽管我们在讨论中选择了右移,但上限可能会也可能不会向右移。Paulson et al. 赞同这一推测,他们认为在低碳酸血症时,上限向右移动,结果是平台被延长。然而,无论是 Paulson *et al.* 还是 Paulson 所引用的两项研究,都没有具体研究低碳酸血症对上限的影响。未来的研究需要解决这个未知领域。
In summary, at hypocapnia, the plateau of the autoregulation curve shifts downward; any change in the lower limit is unremarkable; however, how the upper limit moves is not clear.
总之,在低碳酸血症时,自动调节曲线的平台向下移动;下限的任何变化都不明显;然而,上限如何移动还不清楚。
方法学问题
Methodological Considerations
We have discussed the integrated effect of carbon dioxide and perfusion pressure on the cerebral circulation based on the published large animal and human experimental data and some speculation on the aspects that are without data. Effort has been made to ensure that the available data and the proposed conceptualization do not conflict. Nonetheless, challenges exist due to the differences in the methods used by previous investigators that include study subjects, CPP manipulation, PaCO2 manipulation, CBF measurement, and anesthesia choice (table 1). Data do not automatically deliver concepts or theories, and it is especially unlikely that a single data set could do so. To establish a complete theory, careful data analysis and logical extrapolation are needed in addition to some speculation on the components that are without direct data support.
我们根据已发表的大型动物和人体实验数据以及对没有数据的方面的一些推测,讨论了二氧化碳和灌注压对脑循环的综合影响。我们努力确保现有的数据和提议的概念化不发生冲突。尽管如此,由于以前的研究者所使用的方法不同而存在难度,包括研究对象、CPP 操作、PaCO2 操作、CBF 测量和麻醉选择(表 1)。数据不会自动提供概念或理论,尤其是单个数据集不太可能做到这一点。为了建立一个完整的理论,除了对没有直接数据支持的部分进行一些推测外,还需要仔细的数据分析和逻辑推断。
表 1
Table 1. Details of the Methods Used in the Studies Cited
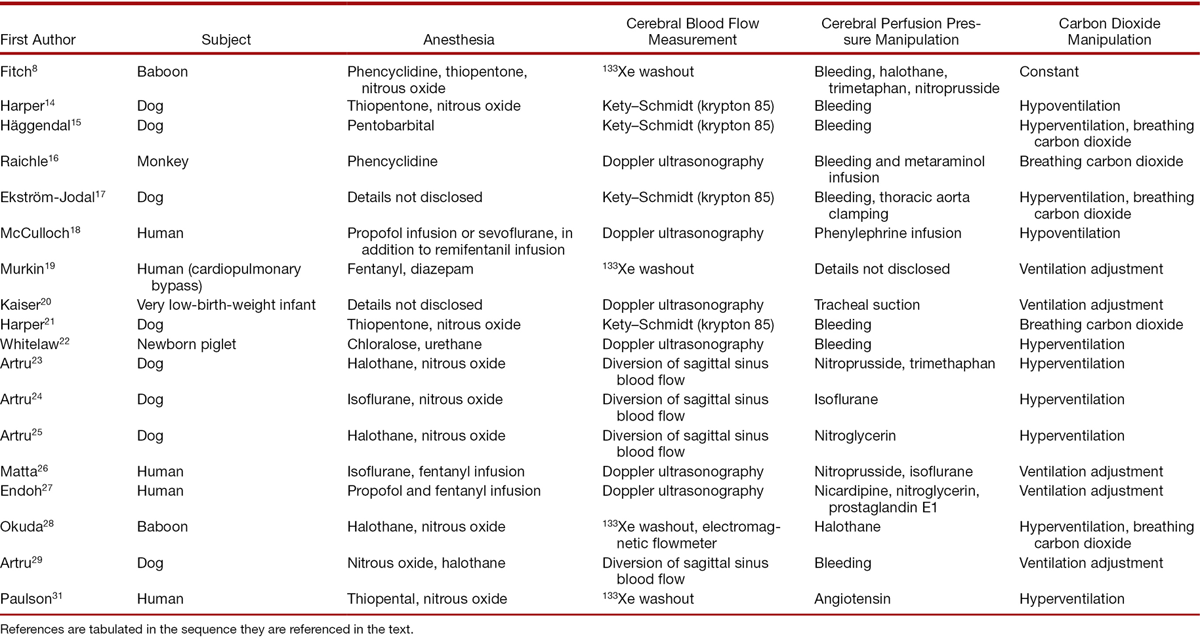
Studies on the relationship between distinct physiological processes can be confounded by unrecognized or unmeasured processes associated with the processes being studied. Sympathetic nervous activity can be a confounder when studying the effect of hypercapnia on cerebral circulation. Busija and Heistad showed that the hypercapnia-induced increase in CBF was further increased by bilateral sympathectomy and attenuated by bilateral electrical stimulation of the superior cervical ganglion in anesthetized cats and unanesthetized rabbits. Cassaglia et al. also reported that the CBF was augmented by sympathetic withdrawal via bilateral superior cervical ganglionectomy during acute hypercapnia in sleeping lambs. Zhang et al. showed that the sensitivity of cerebral vasoreactivity to hypercapnia was attenuated by augmented sympathetic activity induced via lower body negative pressure in healthy young subjects. Moreover, it is known that hypercapnia can increase sympathetic activity alone and with hypoxia. Collectively, this evidence suggests that the hypercapnia-induced increase in CBF is buffered by sympathetic nervous activity. During anesthesia, sympathetic activity is altered, ranging from inhibition to stimulation depending on the anesthetic agent being used, implying that sympathetic activity could confound a study on the effect of hypercapnia on cerebral circulation in anesthetized subjects.
对不同生理过程之间关系的研究可能会被与被研究过程相关的未被认识或未被测量的过程所混淆。在研究高碳酸血症对脑循环的影响时,交感神经活动可能是一个混淆因素。Busija 和 Heistad 表明,在麻醉的猫和未麻醉的兔子中,双侧交感神经切除会进一步增加高碳酸血症引起的 CBF 增加,并通过双侧颈上神经节的电刺激减弱。Cassaglia et al. 也报告说,在睡着的羔羊急性高碳酸血症期间,通过双侧颈上神经节切除,增加了 CBF。Zhang et al. 表明,在健康的年轻受试者中,通过降低身体负压诱导的交感神经活动增强,脑血管活动对高碳酸血症的敏感性减弱了。此外,众所周知,高碳酸血症可单独增加交感神经活动,也可与缺氧一起增加。这些证据共同表明,高碳酸血症引起的 CBF 增加是由交感神经活动缓冲的。在麻醉期间,交感神经活动会发生改变,从抑制到刺激不等,这取决于所使用的麻醉剂,这意味着交感神经活动可能会混淆关于高碳酸血症对麻醉对象的脑循环影响的研究。
Hypocapnia is achieved via hyperventilation in all studies we referenced. However, hyperventilation itself can be a confounder. Alexander et al. showed that hyperventilation caused a consistent increase in blood pressure and decrease in cardiac output in patients anesthetized with propofol and remifentanil. The associated changes in blood pressure and cardiac output can confound studies on the effect of hypocapnia on cerebral circulation due to the influence of systemic circulation on cerebral perfusion.
在我们参考的所有研究中,低碳酸血症是通过过度通气实现的。然而,过度通气本身也可能是一个混淆因素。Alexander et al. 显示,过度通气导致使用丙泊酚和瑞芬太尼麻醉的病人血压持续上升,心输出量下降。由于全身循环对脑灌注的影响,相关的血压和心输出量的变化会混淆关于低碳酸血症对脑循环影响的研究。
A variety of anesthetic agents were used in previous studies (table 1). Type of anesthesia can also be a confounder. It is known that volatile agents cause an increase in CBF due to the intrinsic cerebral vasodilatory property, whereas propofol representing intravenous agents exerts the opposite effect. As a result, volatile agents impair cerebral autoregulation while propofol preserves it, somewhat resembling hypercapnia and hypocapnia, respectively. Therefore, the effect of carbon dioxide on cerebral autoregulation should also be considered in the context of the anesthetic agent being used.
以前的研究中使用了各种麻醉剂(表 1)。麻醉类型也可能是一个混淆因素。众所周知,由于内在的脑血管扩张特性,挥发性药剂会引起 CBF 的增加,而代表静脉注射药剂的丙泊酚则发挥相反的作用。因此,挥发性药剂会损害脑的自动调节功能,而丙泊酚则会保留它, 分别有点类似于高碳酸血症和低碳酸血症。因此,二氧化碳对脑自律调节的影响也应在所使用的麻醉剂的背景下考虑。
We focused our discussion on the integrated effect of carbon dioxide and perfusion pressure on the cerebral circulation. Although it is important to understand the physiology comprehensively, we did not include every aspect that engages in CBF regulation. Oxygen is one of these aspects that deserve emphasis. In 1940s, Kety and Schmidt showed in volunteers that an inspired oxygen fraction of 0.85 to 1.0 was associated with a 13% reduction in CBF, whereas an inspired oxygen fraction of 0.1 produced a 35% increase in CBF. In 1960s, Häggendal and Johansson showed that a decrease in arterial blood oxygen saturation from 90% to 20 to 30% led to an increase in CBF of 100%. Gupta et al. found that the threshold for hypoxic cerebral vasodilation was at a peripheral oxygen saturation of 90% in healthy volunteers, much higher than previously reported. Brown et al. argued that it is the arterial blood oxygen content that is fundamentally important in the regulation of CBF. On the mechanism, adenosine, nitric oxide, cyclic nucleotides, and adenosine triphosphate–sensitive K+ channels are all implicated as being responsible for the hypoxia-induced cerebral vasodilation. On the interplay among oxygen, carbon dioxide, and perfusion pressure on the cerebral circulation, it was found that the cerebrovascular carbon dioxide reactivity was attenuated during acute hypoxia and the impairment of dynamic cerebral autoregulation during isocapnic hypoxia could be prevented with hypocapnia. Conversely, brain tissue oxygen tension is regulated by carbon dioxide and perfusion pressure, resembling the well-known CBF regulation by carbon dioxide and CPP, respectively. This observation reflects that one of the fundamental goals of cerebral perfusion is oxygen delivery. In summary, oxygen regulates CBF both alone and via an integrated mechanism that involves interplay with carbon dioxide, perfusion pressure, and maybe other physiological processes.
我们的讨论集中在二氧化碳和灌注压对脑循环的综合影响。虽然全面了解生理学是很重要的,但我们并没有包括参与 CBF 调节的每一个方面。氧气是其中一个值得强调的方面。20 世纪 40 年代,Kety 和 Schmidt 在志愿者中显示,吸入氧浓度为 0.85 至 1.0 时,CBF 减少 13%,而吸入氧浓度为 0.1 时,CBF 增加 35%。在 20 世纪 60 年代,Häggendal 和 Johansson 表明,动脉血氧饱和度从 90% 下降到 20% 到 30% 会导致 CBF 增加 100%。Gupta et al. 发现在健康志愿者中,缺氧性脑血管扩张的阈值为外周血氧饱和度 90%,比以前的报告要高得多。布朗等人认为,在 CBF 的调节中,动脉血氧含量才是根本性的重要。在机制上,腺苷、一氧化氮、环核苷酸和三磷酸腺苷敏感的 K+ 通道都被认为是负责缺氧引起的脑血管扩张。 关于氧气、二氧化碳和灌注压对脑循环的相互作用,发现在急性缺氧期间,脑血管的二氧化碳反应性减弱,等碳酸血症缺氧期间动态脑血流自身调节的损害可以通过低碳酸血症来防止。 相反,脑组织氧分压受二氧化碳和灌注压的调节,类似于众所周知的 CBF 分别受二氧化碳和 CPP 的调节。 这一观察反映了脑灌注的基本目标之一是氧气输送。总之,氧气既可以单独调节 CBF,也可以通过一个综合机制,包括与二氧化碳、灌注压以及可能的其他生理过程的相互作用。
It needs to be noted that the flat plateau (zero tilt) of the autoregulation curve is likely an idealized drawing. In reality, cerebral autoregulation may execute on a (slightly) tilted plateau that is different to pressure-passive flow. Moreover, the sharp inflection points at both the lower and the upper limits should probably be drawn as a round “shoulder” rather than a sharp “elbow” because the former conforms to normal physiology, whereas the latter is derived as a result of statistical processing.
需要注意的是,自动调节曲线的平坦平台(零倾斜)可能是一种理想化的画法。在现实中,大脑血流自身调节可能在一个(稍微)倾斜的平台上执行,这与压力被动性血流不同。 此外,下限和上限的尖锐拐点可能应该画成一个圆形的 "肩膀" 而不是一个尖锐的 "肘部",因为前者符合正常生理学,而后者是统计处理的结果。
临床意义
Clinical Implications
Cerebral autoregulation is an important mechanism in protecting the brain from ischemia and overperfusion in the face of fluctuating perfusion pressure. As such, it is regularly referenced in clinical practice when taking care of patients with or without neurologic pathophysiologies. However, ignorance of both the limitations and the dynamic/integrative nature of this concept can do more harm than good. The practice of applying a fixed number learned from textbooks or other resources in an individual patient is risky for the following reasons. First, it can either underestimate or overestimate the true value of the lower limit, the upper limit, or the plateau of an individual patient because the commonly quoted numbers are the means of the populations studied without noting the SD or range of distribution. Second, the functional status of cerebral autoregulation is not routinely monitored in clinical care. It can be impaired in a variety of situations such as traumatic brain injury and anesthesia with volatile agents. If so, CBF becomes pressure passive and a different conceptual framework is needed. Finally, nonperfusion pressure conditions or processes, such as carbon dioxide as discussed in this article, can alter the position and shape of the autoregulation curve via their modulating effect on cerebral vasomotor tone. Therefore, cerebral autoregulation is a dynamic process that is regulated by nonperfusion pressure but CBF-regulating aspects.
大脑血流自身调节是保护大脑在面对灌注压波动时免受缺血和过度灌注的重要机制。因此,在临床实践中,在救治有或没有神经系统病理生理学的病人时,经常参考它。然而,对这一概念的局限性和动态 / 整合性质的无知会造成更大的伤害。将从教科书或其他资源中学到的固定数字应用于病人个体的做法是有风险的,原因如下。首先,它可能低估或高估个体病人的下限、上限或平台的真实值,因为通常引用的数字是所研究人群的平均值,而没有注意到 SD 或分布范围。第二,在临床救治中没有对大脑血流自身调节的功能状态进行常规监测。它可以在各种情况下受损,如脑外伤和使用挥发性药剂的麻醉。如果是这样,CBF 就会变成压力被动性,需要一个不同的概念框架。最后,非灌注压条件或过程,如本文讨论的二氧化碳,可以改变自动调节曲线的位置和形状,通过它们对脑血管舒缩张力的调节作用。因此,大脑血流自身调节是受非灌注压调控一个动态过程,调节着 CBF 的各个方面。
The aim of this review is to reanalyze the conceptualization of cerebral autoregulation and not to deal specifically with the relationship between decreased (or increased) blood pressure and neurological outcome. This was recently reviewed. Nonetheless, a pertinent clinical question is what the practical strategy of arterial blood pressure management is when real-time cerebral perfusion and autoregulation are not monitored. Unfortunately, there is no single or simple answer. It depends on the patient’s neurologic pathophysiology including cerebral metabolic need, adequacy of perfusion, intracranial pressure, and integrity of cerebral autoregulation, in addition to the presence of cardiac disease, pulmonary disease, anemia, and so on, as well the largely unknown effects of vasoactive drugs. Clinical care should balance the needs of different organ systems. The complexity of this philosophy is illustrated, for example, with the triple “H” (hypertension, hypervolemia, and hemodilution) therapy that benefits the brain but may harm the heart and the perioperative β-blockade therapy that helps the heart but may hurt the brain. Although the cause–effect role of blood pressure in these dilemmas is hard to define, it is clear that blood pressure management is a decision characterized by priority and balance. The ultimate vindication of any intervention should be based on randomized and controlled trials demonstrating an overall beneficial outcome and this applies equally to the care of the systemic and cerebral circulations. Thus far, large meaningful trials are lacking.
本综述的目的是重新分析脑血流自身调节的概念,而不是具体处理血压降低(或升高)与神经系统结果之间的关系。 尽管如此,一个相关的临床问题是,当不能实时监测脑灌注和自动调节时,动脉血压管理的实际策略是什么。不幸的是,没有单一或简单的答案。它取决于病人的神经系统病理生理学,包括脑代谢需要、灌注充足、颅内压和脑血流自身调节的完整性,此外还有心脏疾病、肺部疾病、贫血等的存在,以及血管活性药物的大部分未知影响。临床救治应平衡不同器官系统的需求。例如,三 "H"(高血压、高血容量和血液稀释)疗法有益于大脑,但可能损害心脏,围手术期 β- 受体阻滞疗法有助于心脏,但可能损害大脑。 尽管血压在这些困境中的因果作用难以界定,但显然,血压管理是一个以优先和平衡为特征的决定。任何干预措施的最终证明都应基于随机和对照试验,证明总体有益的结果,这同样适用于对全身和脑循环的治疗。到目前为止,还缺乏有意义的大型试验。
The clinical implications of the effect of carbon dioxide on cerebral autoregulation are summarized in figure 5. An increase in CBF due to hypercapnia renders the match between CBF and cerebral metabolic rate tilted toward more CBF than needed if cerebral metabolic rate remains unchanged (fig. 2). This may be seen as a safer situation in terms of the maintenance of the supply of cerebral metabolic substrates. However, it needs to be noted that an acute increase in PaCO2 not only shifts the plateau up but also shortens it. A shrunken plateau increases the chance of CBF fluctuation as CPP fluctuates. Under anesthesia, a PaCO2 of greater than 55 mmHg should be regarded as having eliminated autoregulation. Therefore, a tighter CPP control is needed to avoid CBF fluctuation although some “protection” may come from the higher-than-needed CBF (assuming a stable metabolic demand) that would allow a greater decrease in perfusion pressure before ischemia.
图 5 总结了二氧化碳对大脑血流自身调节的影响的临床意义。由于高碳酸血症导致的 CBF 增加,如果脑代谢率保持不变的话,使 CBF 和脑代谢率之间的匹配变为 CBF 的增加超过需求(图 2)。这可以看作是在维持脑代谢底物供应方面比较安全的情况。然而,需要注意的是,PaCO2 的急性增加不仅使平台上移,而且还缩短了它。随着 CPP 的波动,缩减的平台会增加 CBF 波动的机会。在麻醉状态下,PaCO2 大于 55mmHg 应被视为消除了自动调节。 因此,需要更严格的 CPP 控制以避免 CBF 波动,尽管一些 "保护" 可能来自高于需要的 CBF(假设有稳定的代谢需求),允许在缺血前更多地降低灌注压。
图 5
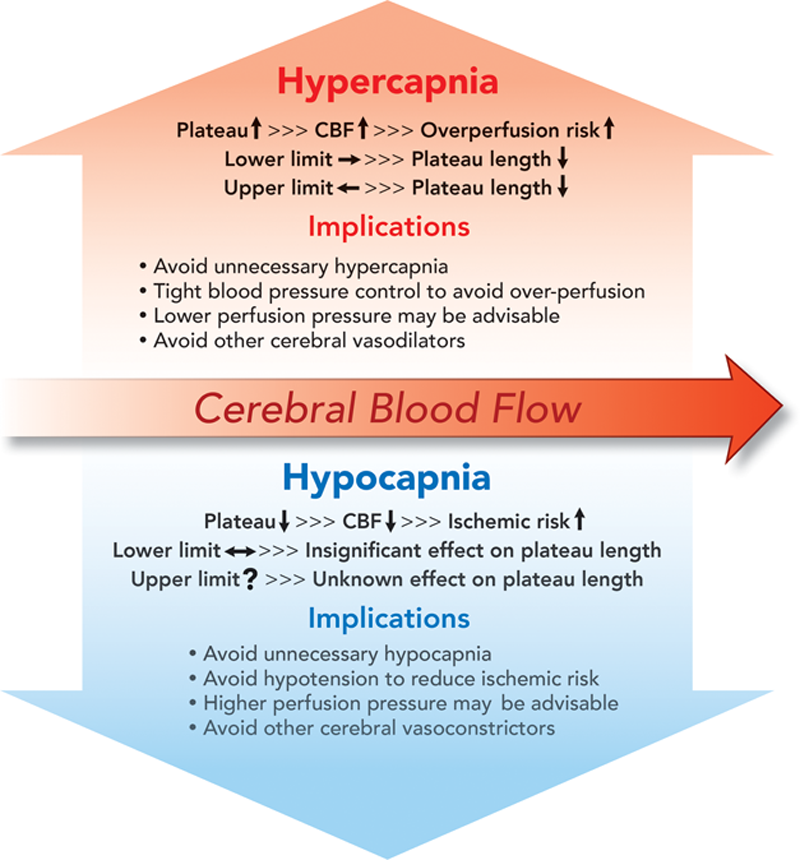
Fig. 5. Clinical implications of the effects of hypercapnia and hypocapnia on cerebral autoregulation. CBF = cerebral blood flow; ↑ = increase or upward shift; ↓ = decrease or downward shift; → = rightward shift; ← = leftward shift; ↔ = insignificant shift.
图 5. 高碳酸血症和低碳酸血症对大脑血流自身调节的影响的临床意义。CBF = 脑血流;↑= 增加或上移;↓= 减少或下移;→= 右移;←= 左移;↔= 不明显的转变。
If cerebral metabolic rate remains unchanged, a decrease in CBF due to hypocapnia renders the brain at risk of cerebral ischemia (figs. 3 and 4). It is true that the cerebrovascular response to hypocapnia is attenuated during hypotension secondary to hemorrhage, drug, or anesthesia. However, this “over-ride” mechanism deals specifically with the effect of hypotension on the cerebrovascular carbon dioxide reactivity, not with the effect of hypocapnia on cerebrovascular pressure reactivity (autoregulation). Studies showed that the CBF was significantly reduced during combined hypocapnia and hypotension., There is no evidence showing that the CBF during combined hypocapnia and hypotension is increased above baseline. Therefore, our cautious recommendation is to avoid hypotension during hypocapnia to decrease the ischemic risk. The decision to implement hypocapnia in clinical care should be weighed against the inherent ischemic risk it incurs. The deleterious effect of hypocapnia in patients with head trauma was reviewed. Hypocapnia was also associated with unfavorable functional outcomes at 90 days after acute stroke.
如果脑代谢率保持不变,由于低碳酸血症引起的 CBF 减少,使大脑面临脑缺血的危险(图 3、4)。诚然,在继发于出血、药物或麻醉的低血压期间,脑血管对低碳酸血症的反应会减弱。然而,这种 "超驰" 机制专门处理低血压对脑血管二氧化碳反应性的影响,而不是低碳酸血症对脑血管压力反应性(自动调节)的影响。研究显示,在合并低碳酸血症和低血压期间,CBF 明显减少。, 没有证据显示在合并低碳酸血症和低血压期间,CBF 高于基线增加。因此,我们谨慎的建议是在低碳酸血症期间避免低血压以减少缺血风险。在临床救治中实施低碳酸血症的决定应与它所带来的固有的缺血风险相权衡。低碳酸血症对头部创伤患者的有害影响已被回顾。 低碳酸血症还与急性卒中后 90 天的不利的功能性预后有关。
总结
Summary
Cerebral autoregulation is a mechanism that maintains CBF stable despite the fluctuation of CPP. As such, it is regularly referenced in clinical care; however, ignorance of its dynamic nature and limitations can do more harm than good. Nonperfusion pressure but CBF-regulating processes such as carbon dioxide affect the efficiency of pressure autoregulation because they intercept at the same target—the cerebrovascular reactivity. The integrated effect of carbon dioxide and perfusion pressure on cerebral circulation is discussed based on the published large animal and human data and some speculation on the aspects that are without data support. We showed that during hypercapnia, the plateau ascends and shortens, the lower limit shifts rightward, and the upper limit leftward. Conversely, during hypocapnia, the plateau descends and the lower limit remains unchanged. How the upper limit is affected by hypocapnia is not clear; nonetheless, we provided two lines of speculation: one line assuming the same calibers of the maximally constricted cerebral resistance vessels at hypocapnia and normocapnia and the other assuming a smaller caliber at hypocapnia than normocapnia.
大脑血流自身调节是一种在 CPP 波动的情况下保持 CBF 稳定的机制。因此,它在临床救治中经常作为参考;然而,对其动态性质和局限性的忽视会造成更大的伤害。非灌注压和 CBF 调节过程(如二氧化碳)会影响压力自动调节的效率,因为它们的靶点是同一个目标 -- 脑血管反应性。根据已发表的大型动物和人类数据以及对没有数据支持的问题的一些猜测,讨论了二氧化碳和灌注压对脑循环的综合影响。我们表明,在高碳酸血症期间,平台上升并缩短,下限向右移动,上限向左移动。反之,在低碳酸血症期间,平台下降,下限保持不变。上限如何受低碳酸血症的影响尚不清楚;尽管如此,我们提供了两种推测:一种是假设低碳酸血症和正常时最大收缩的脑阻力血管口径相同,另一种是假设低碳酸血症时的口径比正常时小。
图表
图 1
图 2
图 3
图 4
图 5
表 1
α 稳态
通常将 pH 稳态的定义描述为不管温度如何变化,均使血液维持在 pH7.35-7.45、PaCO2 35-45mmHg 的状态,维持血液 pH 稳态的酸碱调节方法称为 pH 稳态法。a 稳态理论是 Rahn 等于 20 世纪 70 年代提出的。所谓 a 稳态是指能够保持蛋白质组氨酸 α 咪唑基恒定解离的状态,亦称 “α 咪唑稳态”。α 稳态亦即稳定的蛋白质解离状态。在温度变化时,由于 α 稳态的作用,在一定范围内可以增加或者减少 H 的离解,从而对变温导致的 H 浓度变化起缓冲作用,其最终结果是使细胞外液中 [OH-] 与 [H+] 间的比例关系得以保持稳定,细胞内液 [OH-]/[H+] 比值接近于 1, 细胞外液 [OH-]/[H+] 比值为 15-20,低于 15 为酸中毒,高于 20 为碱中毒。可见,α 稳态的实质是指能够维持 [OH-]/[H+] 比值相对稳定的状态,它不受温度的影响。维持血液 α 稳态的酸碱调节方法称为 α 稳态法。
参考文献